an email newsletter released every month highlighting the latest articles, events, technical inquiries, and voices from the community
Microreactor Technology
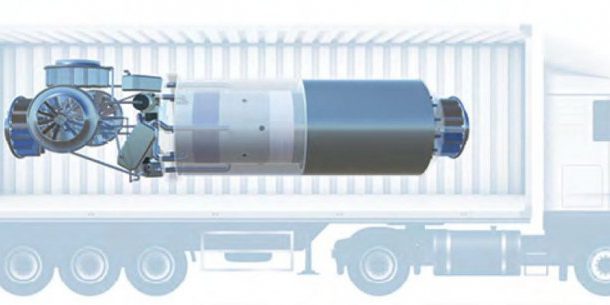
Posted on December 30, 2022 | Completed on January 12, 2022
Can you research and provide publicly releasable microreactor power information geared toward supporting U.S. military efforts that have dual-use capabilities?
Microreactor technology has the potential to provide benefits to the military in both domestic and overseas operations. In response to a technical inquiry received by the Homeland Defense and Security Information Analysis Center, several microreactor programs were explored. This includes three government programs: (1) Project Pele, sponsored by the U.S. Department of Defense, (2) the Microreactor Applications Research Validation and Evaluation Project, and (3) Modeling-Enhanced Innovations Trailblazing Nuclear Energy Reinvigoration, which are both sponsored by the U.S. Department of Energy. Some commercial technologies are included as well. While there are supply chain and technology issues to overcome, microreactors are showing promise as a power-generation technology.
1.0 TI Request
1.1 Inquiry
A request was submitted to research and provide microreactor power information that is publicly releasable and geared toward support for U.S. military efforts that have dual-use capabilities.
1.2 Description
The requestor wanted to focus the research on information from government programs for microreactors that may have dual-use capabilities. The primary use case would be humanitarian assistance/disaster relief efforts.
2.0 TI Response
The Homeland Defense & Security Information Analysis Center (HDIAC) leaned on previous research regarding microreactors to jump-start the research for the current inquiry. HDIAC reviewed documents from current projects known to be underway by the government and searched open-source information provided by industry developers and manufacturers of microreactor technology. Initial information was provided to the inquirer, while a deeper dive and analysis are provided in this report.
3.0 Overview
The idea of small modular reactors (SMRs) and microreactors is not new. “Established in 1954, the Army Nuclear Power Program (ANPP) was a joint effort between the U.S. Army Corps of Engineers and the Atomic Energy Commission to develop nuclear power plants for military use” [1]. This program developed many small-scale prototypes. Most notably, Argonne National Laboratory designed and built the Stationary Low Power Reactor No. 1 (SL-1) in the late 1950s. “The SL-1 was a direct cycle, natural recirculation boiling water reactor designed for 3,000 kW gross thermal capacity and was capable of producing 200 kW net of electricity and 1.3 million BTU per hour for space heat” [2]. Unfortunately, SL-1 would suffer a critical meltdown on 3 January 1961, killing the three military operators. While the ANPP would continue, it was ultimately shut down in 1976 [1]. Recently, the concept of SMRs and microreactors resurged and has gained ground. In particular, microreactors provide many potential benefits over both large commercial nuclear reactors and SMRs. Large commercial reactors can provide more than 300 megawatts electric (MWe) of power. SMRs have a capacity of 20–300 MWe, while microreactors produce less than 20 MWe. Large commercial reactors generally take years of planning, site preparation, and onsite construction before they can begin power production. They are also fixed in a single location, whereas microreactors are able to be moved to different locations as needed [3].
A major benefit to microreactors over both large commercial reactors and SMRs is their reproducibility and standardization. “Microreactors are on the other end of the spectrum from [gigawatt]-scale reactors, with factory fabrication, quick installation, and economy of multiples replacing the multiyear field construction and economy of scale approach” [3]. Domestic applications for microreactors are growing as the community is beginning to realize their viability. The military is also viewing microreactors as a potential way to reduce the logistical fuel needs of operating in a forward environment.
Figure 1 shows the “hub-spoke” model for domestic employment of microreactors, with a factor sitting as the hub and several use cases (remote communities, remote mining, energy demand points, and industry applications) serving as the spokes.
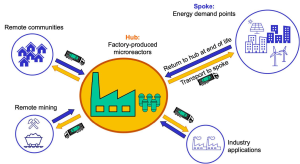
Figure 1. Illustration of the “Hub-Spoke” Model for Fabricating and Deploying Microreactors [3].
Pairing microreactors with intermittent clean energy sources could provide uninterrupted, clean power to a microgrid.
Several government-funded projects stand out, particularly Project Pele and the Microreactor Applications Research, Validation, and Evaluation Project (MARVEL). Microreactors could provide sustainable, clean, and uninterrupted power to a small-scale grid. They could be placed in remote areas to provide electricity and heat without being refueled for years.
3.1 Project Pele
On 27 January 2021, the President signed Executive Order 14008, “Tackling the Climate Crisis at Home and Abroad.” This order states “that climate considerations shall be an essential element of United States foreign policy and national security” [4]. In response, the “[U.S. Department of Defense] DoD commissioned the Defense Science Board to study alternative energy technologies for forward operating bases, remote operating bases, and expeditionary forces” [5]. This was the first step on a road that has led, so far, to Project Pele.
“Supplying liquid fuel to military forces is a significant challenge, as the commodity typically comprises a large portion of the mass transported to deployed locations” [5]. Throughout the long military deployments in Afghanistan and Iraq, most power was provided through diesel-fired generators. This left a long logistical tail for fuel, as well as the need for constant monitoring and maintenance. In Fiscal Year (FY) 2007, Operation Iraqi Freedom consumed 617 million gallons of fuel and Operation Enduring Freedom consumed 153 million gallons [6]. While it is not clear how much of that fuel was used for power generation, it can be assumed that reducing the amount of power produced from diesel generators would significantly reduce the amount of fuel consumption. This, in turn, would reduce the need for fuel deliveries, further reducing fuel and transportation costs as well as personnel requirements.
With this backdrop, the DoD finds it not just an advantage but a military necessity to develop an alternative means of powering the force.
3.1.1 Design
The design of Project Pele is not finalized. However, recent documentation describes it as “a high-temperature, gas-cooled reactor” [5]. This is similar to current commercial nuclear reactors in use today, but the Project Pele microreactor would be transportable by ship, rail, truck, or plane.
The DoD has identified several technical requirements for the Project Pele microreactor, as represented in Table 1.
Table 1. Technical Requirements and Objectives of a Mobile Microreactor [5]
Technical Requirement | Technical Objective |
---|---|
Life | Able to generate threshold power (1–10 MWe of electric power generation) for more than 3 years without refueling. |
Wrap Up | Time for planned shutdown, cool down, disconnect, prepared transport, and safe transport: less than 7 days. |
Startup | Time from arrival of unit to reaching full electric power operations: less than 72 hours. |
Size | All components should fit in ISO 688 certified 20- or 40-ft CONEX containers. Government preference is to use 20-ft standard CONEX containers. |
Operation | Semi-autonomous operation (i.e., does not require manned control by operators to ensure safe operation). Minimal manning to monitor overall mobile microreactor and power plant system health. Minimal routine preventative maintenance and repair required. |
3.1.2 Requirements
The DoD has outlined requirements for the type of fuel, reactor core, reactor, power conversion system, and safety. While a deep exploration of each requirement is outside of the scope of this report, the fuel requirements are an important consideration for the use of a microreactor that may be used in a tactical environment. Project Pele uses tristructural isotropic (TRISO) fuel. This is “a transformational technology that allows for inherently safe reactors that can withstand external attacks without creating a large evacuation zone” [7]. The key is finding a fuel that can remain safe even if the microreactor itself has been compromised. This would allow for use in less permissive environments.
Each TRISO particle is made up of a uranium oxycarbide (a mixture of uranium dioxide and uranium carbide) fuel kernel encapsulated by three layers of carbon- and ceramic-based (silicon carbide) material. Each particle acts as its own containment system because of its triple-coated layers. This allows them to retain fission products. The particles are incredibly small (about the size of a poppy seed) and very robust. TRISO fuels are structurally more resistant to neutron irradiation, corrosion, oxidation, and high temperatures (the factors that most impact fuel performance) than traditional reactor fuels.[5]
The “innovative pellet design…is a paradigm shift in safety for nuclear power” [7]. As the safety of the design, particularly the fuel, is increased, the microreactor can be brought closer and closer to the “front lines.” Figure 2 shows a cross-section of a TRISO particle.
3.1.3 Future
The 2019 National Defense Authorization Act directs the U.S. Department of Energy (DOE) and DoD to site, construct, and operate at least one microreactor at a DoD facility by the end of 2027 [9]. According to budget justification documents provided to Congress in 2022, “Modeling will be performed to study the survivability of the designs under adverse conditions, including kinetic” [10]. FY 2023 plans include construction of the prototype transportable nuclear microreactor, fabrication of the TRISO pellets, and study of operational doctrine and training concepts.
The DoD has projected a need of nearly $131 million in FY 2022, with an increase to approximately $226 million in FY 2023. Future projections level off somewhat but increase steadily until FY 2027, where the projected budget is $248 million. This is based on Budget Activities 6.3 and 6.4 justifications [10, 11]. The DoD “has picked BWX Technologies’ microreactor design for its ‘Project Pele’ full-scale transportable prototype…The prototype will be built under a cost-type contract valued at approximately $300 million” [12].
“The [DoD] uses some 30-terawatt hours of electricity annually and more than 10 million gallons of fuel each day and expects those levels to increase. ‘A safe, transportable nuclear reactor would address this growing demand with a resilient, carbon-free energy source that would not add to the DoD’s fuel needs, while supporting mission-critical operations in remote and austere environments,’” the department said in a 13 April [2022] press release [13].
As of February 2023, Project Pele is in its final stages of design. Pending design approval, the project will begin construction with the hope of having the reactor turned on in 2025 at Idaho National Laboratory (INL). Project Pele, and microreactors in general, could be a game changer for the DoD’s energy needs at forward operating bases, along with increasing resiliency and decreasing costs at domestic installations.
3.2 Project MARVEL
Where Project Pele is DoD led, Project MARVEL is DOE led. Specifically, INL has the primary development role. It is important to understand that MARVEL’s purpose “is to meet [research and development] R&D needs identified by [the] DOE and the advanced reactor stakeholder community by designing and building a nuclear microreactor application test platform” [14]. Project MARVEL is being designed as a test reactor. It is not intended to immediately solve a commercial or military problem.
3.2.1 Fuel
Project MARVEL will use training, research, isotopes, general atomics (TRIGA) fuel material [9]. The TRIGA fuel has unique safety features that “makes unnecessary the expensive pressure containment building required by present safety regulations for research reactors” [15]. These unique safety features make TRIGA ideal for microreactors, as the reactor can be brought to a much smaller area.
3.2.2 Future
In a briefing in March 2022, INL provided the next steps for calendar year 2023. They included fuel production, fuel load, and criticality, as well as achieving the MARVEL microgrid [16]. This will be a major victory for the future of microreactors; Project Pele and other commercially developed systems will rely on lessons learned from Project MARVEL.
3.3 Modeling-Enhanced Innovations Trailblazing Nuclear Energy Reinvigoration (MEITNER)
MEITNER is a program led by the Advanced Research Projects Agency—Energy (ARPA-E). Created in 2018, MEITNER seeks “to identify and develop innovative technologies that can enable designs for lower cost, safer, advanced nuclear reactors” [17].
There are multiple designs being considered under MEITNER. ARPA-E selected HolosGen to design a transportable modular reactor for the MEITNER project using TRISO fuel. Unlike current large reactors with only baseload capability, the HolosGen-designed reactor will have load-following ability. This will allow the amount of power output to change rapidly as other sources, particularly intermittent wind and solar sources, change their outputs [18]. ARPE-E also funded Moltex Energy to develop composite structural technologies for its stable salt
reactor. “This will bring the Stable Salt Reactor-Wasteburner (SSR-W)…even closer to market and global roll-out” [19].
The MEITNER program consists of several other supporting projects to develop and commercialize microreactors. This includes a North Carolina State University project to design a management and control system for advanced reactors; a Yellowstone Energy project for a
reactivity control device for advanced reactors; and a University of Illinois, Urbana-Champaign project to develop a fuel-processing system that enables load following in molten salt reactors [17].
Enabling load following is a critical element in the design and employment of microreactors. Traditional large nuclear reactors are baseloading power plants. According to the International Atomic Energy Agency (IAEA), a baseload operation is the “operation of a power station or generating unit at steady full load as far as possible, and not load following or providing automatic frequency control” [20]. This means that a power plant will operate at a steady rate of output with very little fluctuation. Traditional nuclear plants typically follow this model and can take hours to change their output, therefore making it much more economical to have them operate as a baseload power plant.
On the other hand, load following is defined by the IAEA as “varying the output of a generating unit in a planned way or in response to an instruction or control signal from the grid control centre” [20]. This would allow for changes in electrical output as energy demand changes. This will be a critical development to allow a microreactor to operate independently on a microgrid. It would also allow microreactors to be coupled with intermittent renewable energy sources.
Project MARVEL is currently forecasting a completion date of the fourth quarter of calendar year 2024.
3.4 Other Efforts
There are commercial efforts to design and develop microreactors commercially. Discussed next are some efforts taking place in the United States.
3.4.1 Westinghouse
Westinghouse is designing a microreactor called eVinci. According to their website: “The key benefits of the eVinci™ microreactor are attributed to its solid core and advanced heat pipes. The heat pipes enable passive core heat extraction, allowing autonomous operation and
inherent load-following capabilities. These advanced technologies together make the eVinci™ microreactor a pseudo ‘solid-state’ reactor with minimal moving parts.” Some of the attributes of the eVinci reactor are [21]:
• Transportable energy generator
• Fully factory built, fueled, and assembled
• Combined heat and power delivery: 5 MWe and up to 13 MW
• 8+ years of full-power operation prior to refueling
• Target less than 30 days on-site installation
• High-speed load-following capability
• High reliability and minimal moving parts
• Autonomous operation capability
• Near-zero emergency planning zone with small site footprint
• No spent fuel or waste storage on site
• Simplified decommissioning and remediation
3.4.2 X-Energy
X-Energy, LLC is designing a reactor with carbon-free emissions that can be deployable to meet the expected energy demand anywhere worldwide [22].
The following lists information on the X-Energy reactors:
X-Energy XE Mobile Reactor [23]:
o Transportable nuclear electricity production
o Rapid deployment and redeployment goal
o Safe use over product lifecycle
o Reliable, consistent, and semi-autonomous operations
o No construction or site prep required
o Transportable by rail, truck, and U.S. military aircraft
o Components housed in ISO containers
o Full-power operation capability for longer than 3 years
o High maturity level and strong safety case for TRISO fuel source
o 1-MWe+ of electrical power production
o Ability to choose voltage output
X-Energy Xe-100 Reactor [24]:
o Gen-IV high-temperature gas-cooled reactor
o 80-MWe individual reactor with scalability into a 320-MWe four-pack power plant
o 60-year operational life design
o American-Society-of-Mechanical-Engineers-compliant reactor vessel, core barrel,
and steam generator
o Modularability to allow for easy transportation, scalability, and cost control
o 400-yd safety perimeter (vs. 10-mile safety perimeter when compared with other
Gen-IV reactors)
o Meltdown-proof safety design
3.4.3 NuScale
The NuScale microreactor is being designed to provide 10–50 MWe of power and a small 1–10-MWe heat pipe reactor. Their designs boast a 10-year cycle before needing to be refueled. “Of note are the current plans (agreement is under development) among the DOE, the cooperative utility Utah Associated Municipal Power System, and INL to deploy the first-of-a-kind NuScale plant at INL. The first deployment…is projected for commercial operation in 2026” [25].
The NuScale reactor includes several redundant safety features and a safety standoff significantly less than current large nuclear power programs [25].
3.4.4 Ultra Safe Nuclear Corporation (USNC) Micro Modular Reactor (MMR)
The MMR is designed to generate 5–10 MWe of electricity and 15–30 megawatts thermal (MWth) energy. MMR units can be linked together to provide as much power as needed, and the reactor uses no water, reducing logistical needs. It will have a load-following capability, making it useful to pair with renewable energy sources.
The MMR will use TRISO fuel encased within a fully dense silicon carbide matrix. This creates
a very stable fuel source and temperature stability. USNC boasts several other safety features,
including:
• Low-power density and high surface area that dissipates heat without active measures
• Reduced chemical hazards with all materials and coolants that are noninteracting
• Walk-away safe reactor
• Buried reactor that is safe from accidents or terrorist attacks [26]
3.5 Concerns
The development of more nuclear reactors of any type raises concerns. This includes concrete concerns such as fuel supply and nuclear waste disposal. Discussed next are a couple of those tacit concerns. Public perception of nuclear materials and energy generation is another major concern that must be kept in mind but will not be addressed here.
3.5.1 High-Assay Low-Enriched Uranium (HALEU) Fuel Supply
Supply chain issues are a concern for the development of a fleet of microreactors, but one that can be overcome, particularly with the involvement of the DOE. The main supply question centers around the production of HALEU. HALEU is the primary fuel in most microreactor designs. Russia is the primary producer of HALEU in the world and “is currently the world’s only viable commercial supplier” [27]. Obviously, this is not an ideal situation given the current political situation.
This is not news to the DOE or the nuclear energy community at large. However, there is some concern that the HALEU needs of the developers of new microreactors and other advanced reactors will outpace the supply. “Everett Redmond, a senior technical advisor at [the Nuclear Energy Institute], told Neutron Bytes…that 20 metric tonnes uranium (MTU) are needed by 2025.” According to Redmond, “As things stand now, the HALEU fuel that the reactors need won’t become available until mid-2028” [28].
It is not too late to correct the course on HALEU production. While some in the industry have complained about a lack of forward progress on licensing and policy regarding HALEU production, the DOE maintains that it is working diligently to clear the road for HALEU production. A DOE agency spokesman said the following in response to a question from Neutron Bytes on the development of a more comprehensive uranium strategy:
The [DOE] team focused on the comprehensive uranium strategy is working with a clear sense of urgency to identify options that will meet commercial and DOE uranium needs in the near term, midterm, and long term. This strategy has the mutual objectives of expanding our domestic fuel cycle supply capacity and limiting our dependence on Russian-supplied uranium. An initiative of this magnitude requires extensive input, coordination, and endorsement, so a precise timetable cannot be provided [28]. It appears that DOE is taking an active role in solving the supply chain issues with HALEU fuel production. This will be critical for near-, mid-, and long-term success of advanced nuclear reactors.
3.5.2 Nuclear Waste Management
Nuclear waste is at the forefront of the public’s mind any time the prospect of a new nuclear power plant is contemplated. With the emergence of new advanced reactors and the possibility of greatly expanding the nuclear-generating capability of the United States, these concerns must be addressed before forward progress can be made.
According to the Government Accountability Office, “The nation has over 85,000 metric tons of spent nuclear fuel from commercial nuclear power plants. [The] DOE is responsible for disposing of this high-level waste in a permanent geologic repository but has yet to build such a facility because policymakers have been at an impasse over what to do with this spent fuel since 2010” [29]. There are major issues to be overcome with nuclear waste management that must be addressed before microreactors can become the wave of the future.
4.0 Conclusion
Microreactors are poised to provide electricity in a clean, economical way to add power to large grids or power microgrids in remote areas, islands, and temporary facilities such as military forward operating bases. This technology promises to provide massive changes in U.S. power generation and energy surety.
References
[1] U.S. Army Corps of Engineers. “Army Nuclear Power Program, 1954–1976.” U.S. Army Corps of Engineers Headquarters Website, https://www.usace.army.mil/About/History/Exhibits/Nuclear-Power-Program/, accessed 21 June 2022.
[2] Tardiff, A. N. “Some Aspects of the WTR and SL-1 Accidents.” International Atomic Energy Agency Symposium on Reactor Safety, Vienna, Austria, 14–18 May 1962, https://www.osti.gov/servlets/purl/4828615, accessed 22 June 2022.
[3] Abou-Jaoude, A., Y. Arafat, B. Dixon, and A. Foss. “An Economics-by-Design Approach Applied to a Heat Pipe Microreactor Concept.” Idaho National Laboratory, Idaho Falls, ID, https://inldigitallibrary.inl.gov/sites/sti/sti/Sort_46104.pdf, accessed 28 July 2022.
[4] The White House. “Executive Order on Tackling the Climate Crisis at Home and Abroad.” EO 14008, Washington, DC, https://www.whitehouse.gov/briefing-room/presidentialactions/2021/01/27/executive-order-on-tackling-the-climate-crisis-at-home-and-abroad/, accessed 22 June 2022.
[5] United States Department of Defense. “Final Construction and Demonstration of a Prototype Mobile Microreactor Environmental Impact Statement.” Final Environmental Impact Statement, Strategic Capabilities Office, Washington, DC, February 2022.
[6] Defense Energy Support Center. “Fact Book FY08.” 31st edition, Fort Belvoir, VA, n.d.
[7] Waksman, J. “Project Pele Overview: Mobile Nuclear Power for Future DoD Needs.” https://gain.inl.gov/GAINEPRINEI_MicroreactorProgramVirtualWorkshopPres/Day-2%20Presentations/Day-2-am.02-Nichols_PeleProgOverviewPublicMarch2020,19Aug2020.pdf, accessed June 2022.
[8] Davenport, M., D. A. Petti, and J. Palmer. “Status of TRISO Fuel Irradiations in the
Advanced Test Reactor Supporting High-Temperature Gas-Cooled Reactor Designs.” 2016 International Topical Meeting on High-Temperature Reactor Technology, Las Vegas, NV, 6–10 November 2016.
[9] U.S. Congress. “John S. McCain National Defense Authorization Act for Fiscal Year 2019.” H.R. 5515, Washington, DC, https://www.congress.gov/115/bills/hr5515/BILLS-115hr5515enr.pdf, accessed 28 June 2022.
[10] Office of the Secretary of Defense. “RDT&E Project Justification: Program Element 0604250D8Z/Advanced Innovative Technologies.” Washington, DC, April 2022.
[11] Office of the Secretary of Defense. “RDT&E Project Justification: Program Element 0604055D8Z/Operational Energy Capability Improvement (OECI).” Washington, DC, April 2022.
[12] Patel, S. “DOD Picks BWXT Design for ‘Project Pele’ Prototype Nuclear Microreactor.” Power Magazine, 9 June 2022.
[13] Albon, C. “Pentagon Chooses Design for ‘Project Pele’ Portable Nuclear Reactor Prototype.” DefenseNews, https://www.defensenews.com/battlefieldtech/2022/06/09/pentagon-chooses-design-for-project-pele-portable-nuclear-reactorprototype/#:~:text=The%20U.S.%20Department%20of%20Defense’s,June%209%20that%20the%20Pentagon, accessed 29 July 2022.
[14] United States Department of Energy. “Final Environmental Assessment for the Microreactor Applications Research, Validation, and Evaluation (MARVEL) Project at Idaho National Laboratory.” DOE/EA-2146, Idaho Operations Office, Idaho Falls, ID, June 2021.
[15] General Atomics. “TRIGA Advantages.” General Atomics, https://www.ga.com/triga/advantages, accessed 23 June 2022.
[16] Jackson, J. “Microreactor Program FY2022: Winter Program Review March 3–4th, 2022”. Idaho National Laboratory, Idaho Falls, ID,
https://gain.inl.gov/SiteAssets/MicroreactorProgram/2022MicroreactorProgramWinterReview/02-MRP_FY22%20Winter%20Program%20Review-Jackson.pdf, accessed 29 June 2022.
[17] Advanced Research Projects Agency—Energy. “Modeling-Enhanced Innovations Trailblazing Nuclear Energy Reinvigoration.” arpa-e, https://arpae.energy.gov/technologies/programs/meitner, accessed 29 July 29 2022.
[18] Advanced Research Projects Agency—Energy. “Transportable Modular Reactor.” arpa-e, https://arpa-e.energy.gov/technologies/projects/transportable-modular-reactor, accessed 29 July 2022.
[19] World Nuclear News. “U.S. Federal Funding Granted for SSR Technology Development.” wnn: World Nuclear News, https://world-nuclear-news.org/Articles/US-federal-funding-grantedfor-SSR-technology-deve, accessed 1 August 2022.
[20] International Atomic Energy Agency. “Non-Baseload Operation in Nuclear Power Plants: Load Following and Frequency Control Modes of Flexible Operation.” IAEA Nuclear Energy Series, no. NP-T-3.23, https://www-pub.iaea.org/MTCD/Publications/PDF/P1756_web.pdf,
accessed 1 August 2022.
[21] Westinghouse Electric Company LLC. “eVinciTM Microreactor.” Westinghouse, https://www.westinghousenuclear.com/new-plants/evinci-micro-reactor, accessed 26 June 2022.
[22] X Energy, LLC. “Why? Zero Emissions—We Must Act Now.” X energy, https://xenergy.com/why/zero-emissions, accessed 9 August 2022.
[23] X Energy, LLC. “Xnenergy’s Reactor: Xe-Mobile—A Factory-Built and Fueled Mobile Microreactor for Remote Locations and Microgrids.” X energy, https://x-energy.com/reactors/xemobile, accessed 9 August 2022.
[24] X Energy, LLC. “X-energy’s Xe-100: The Moste Advanced Small Modular Reactor—The Safest, Most Economic and Most Advanced Design for a Small Modular Nuclear Reactor.” X energy, https://x-energy.com/reactors/xe-100, accessed 9 August 2022.
[25] Ingersoll, D. T., and M. D. Carelli. “Small Modular Reactors (SMRs): The Case of the United States of America.” Handbook of Small Modular Nuclear Reactors, pp. 521–553, Sawston, Cambridge: Woodhead Publishing, 2021.
[26] Ultra Safe Nuclear Corporation. “Micro Modular Reactor Energy System.” Ultra Safe Nuclear, https://usnc.com/mmr-energy-system/, accessed 23 August 2022.
[27] Third Way. “Developing Domestic HALEU Supply Spells Freedom From Russian Dependency.” Third Way, https://www.thirdway.org/memo/developing-domestic-haleu-supplyspells-freedom-from-russian-dependency, accessed 11 August 2022.
[28] Yurman, D. “What’s the Holdup with the HALEU Fuel Supply Chain?” Neutron Bytes, https://neutronbytes.com/2022/07/19/whats-the-holdup-with-the-haleu-fuel-supply-chain/, accessed 11 August 2022.
[29] United States Government Accountability Office. “Nuclear Waste Disposal”. GAO, https://www.gao.gov/nuclear-waste-disposal, accessed 12 August 2022.
Want to find out more about this topic?
Request a FREE Technical Inquiry!